Home>Gardening News and Trends>Latest News>1. How Is The Gas Exchange System In Insects Different From The Gas Exchange System In Mammals?
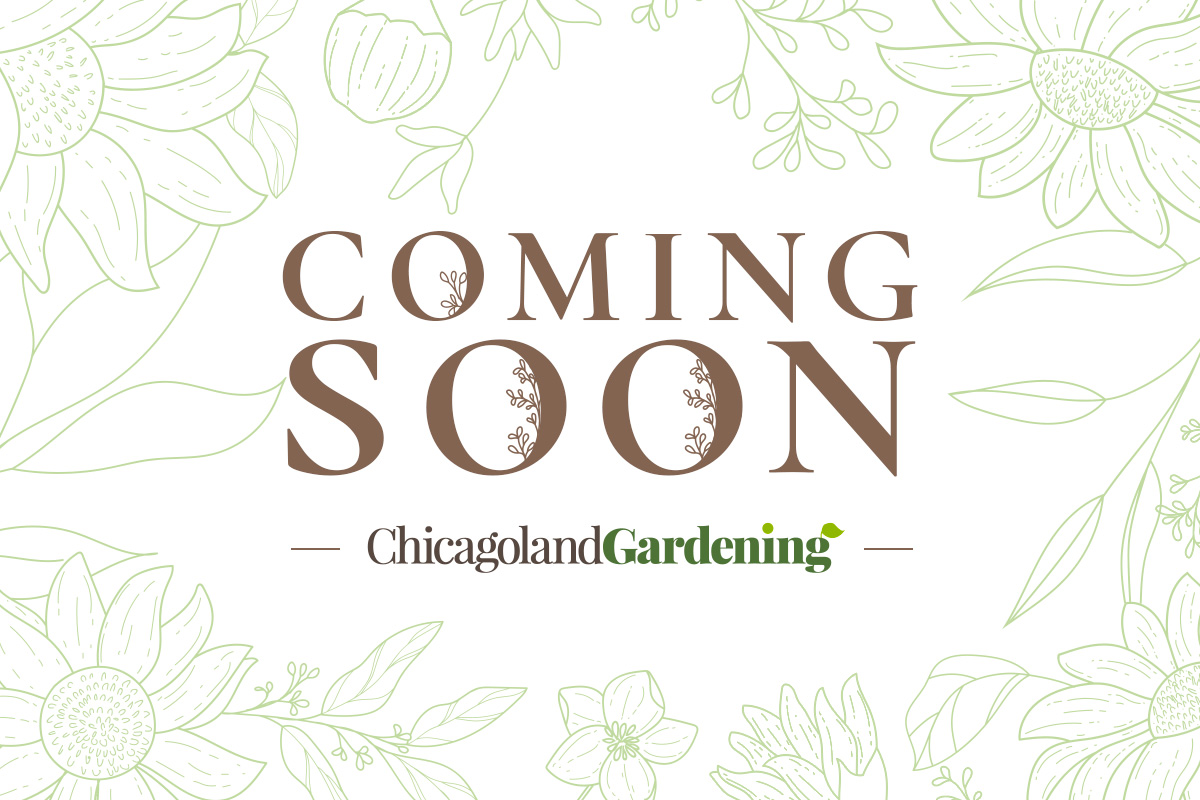
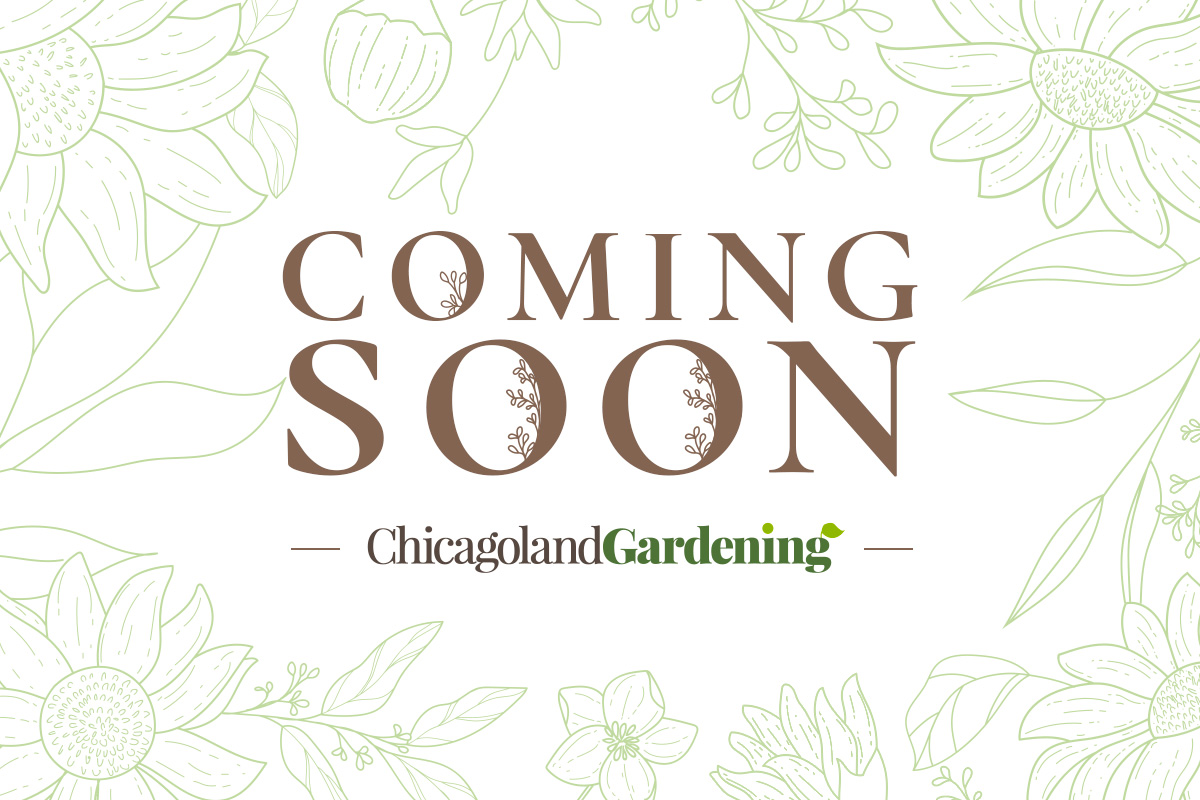
Latest News
1. How Is The Gas Exchange System In Insects Different From The Gas Exchange System In Mammals?
Modified: January 22, 2024
Discover the key differences between gas exchange systems in insects and mammals. Stay updated with the latest news on this fascinating topic.
(Many of the links in this article redirect to a specific reviewed product. Your purchase of these products through affiliate links helps to generate commission for Chicagolandgardening.com, at no extra cost. Learn more)
Table of Contents
- Introduction
- Anatomy of the Gas Exchange System in Insects
- 1 Tracheal System
- 2 Spiracles
- 3 Tracheoles
- Gas Exchange Mechanism in Insects
- 1 Diffusion
- 2 Movement of Gases
- Compare and Contrast with Mammalian Gas Exchange System
- 1 Respiratory Organs
- 2 Lung Structure
- 3 Breathing Mechanism
- Adaptations of Insect Gas Exchange System
- 1 Efficient Oxygen Delivery
- 2 Conservation of Water
- 3 Rapid Gas Exchange Rate
- Conclusion
Introduction
The gas exchange system is a vital process in all organisms, allowing them to obtain oxygen from the environment and expel carbon dioxide. While mammals, including humans, have a well-known respiratory system centered around lungs and blood vessels, insects have developed a unique and highly efficient gas exchange system to meet their oxygen needs. The anatomy and mechanism of gas exchange in insects differ significantly from those of mammals, providing fascinating insights into the diverse adaptations seen in the animal kingdom.
Insects are a diverse class of organisms, comprising over a million known species, and they play crucial roles in ecosystems as pollinators, decomposers, and predators. Their small size necessitates a highly efficient and compact gas exchange system to ensure the delivery of oxygen to all cells and removal of carbon dioxide from their bodies.
This article explores the differences between the gas exchange systems in insects and mammals, highlighting the unique features of the insect respiratory system and giving insights into the adaptations that have evolved to meet their physiological needs. We will delve into the anatomy of the insect gas exchange system, the mechanisms of gas exchange, and a comparison with the mammalian respiratory system.
Understanding how insects exchange gases is not only fascinating but also vital for appreciating their ecological importance and the intricate ways in which they have evolved to survive in diverse environments. By unraveling the complexities of insect physiology, we can gain a greater appreciation for the remarkable adaptations that allow these small creatures to thrive in their respective niches.
Anatomy of the Gas Exchange System in Insects
The gas exchange system in insects is characterized by several unique anatomical structures that facilitate efficient oxygen uptake and carbon dioxide release. These structures include the tracheal system, spiracles, and tracheoles.
The tracheal system is a network of tubes that extends throughout the insect’s body, delivering oxygen directly to cells. Unlike mammals, insects lack specialized respiratory organs like lungs. Instead, their entire body is equipped with a complex network of tracheae, which are supported by chitin, a rigid substance found in their exoskeletons. This system allows for direct gas exchange between the environment and the cells, eliminating the need for a circulatory system to transport gases.
Spiracles are small openings found on the exoskeleton of insects, serving as the entrance and exit points for gases. These tiny openings can be opened and closed by specialized muscles, enabling insects to regulate gas exchange and prevent water loss. The number and placement of spiracles vary among different insect species, but they are usually located on the thorax and abdomen.
Connected to the tracheae are tracheoles, which are even smaller tubes that penetrate into individual cells, ensuring oxygen delivery to every tissue. These tracheoles have thin walls and are in close proximity to cells, allowing for efficient diffusion of gases. The movement of gases in and out of cells occurs through diffusion, driven by concentration gradients.
This intricate network of tracheae, spiracles, and tracheoles enables insects to obtain oxygen directly from the atmosphere and deliver it to their cells. The absence of lungs in insects results in a compact and efficient gas exchange system, well-suited to their small size and high metabolic demands.
1 Tracheal System
The tracheal system is a fundamental component of the gas exchange system in insects. It consists of a vast network of tubes called tracheae, which extend throughout the insect’s body and deliver oxygen directly to the cells.
The tracheae are lined with a thin layer of cuticle, which is impermeable to gases. This prevents gas leakage and allows for efficient gas transport. The tracheae are supported by chitin, a rigid substance found in the exoskeleton of insects. This support provides structural integrity to the tracheal system, allowing it to withstand the pressures associated with respiration.
The tracheae branch repeatedly, forming smaller tubes known as tracheoles. These tracheoles penetrate the insect’s tissues, bringing oxygen directly to the cells and removing carbon dioxide. Tracheoles have extremely thin walls, which facilitate the exchange of gases through diffusion.
The tracheal system in insects is highly efficient, enabling rapid gas exchange. The branching structure of the tracheae ensures that oxygen can reach even the deepest tissues, guaranteeing oxygen delivery to every cell in the insect’s body.
Spiracles, small openings on the surface of the insect’s exoskeleton, serve as the entrance and exit points for gases. They are connected to the tracheae and allow for gas exchange between the environment and the tracheal system. The number and placement of spiracles vary among different insect species, but they are typically found on the thorax and abdomen.
In some insects, such as aquatic larvae, specialized structures called plastrons can be found. Plastrons are thin layers of air held against the body surface by hydrophobic hairs. They function as an additional method for gas exchange, allowing insects to extract oxygen from water and enabling them to live in environments with reduced oxygen levels.
The tracheal system in insects is a remarkable adaptation that allows for efficient oxygen delivery and carbon dioxide removal. By eliminating the need for specialized respiratory organs like lungs, insects have developed a unique and highly effective gas exchange system perfectly suited to their small size and metabolic requirements.
2 Spiracles
Spiracles are small openings found on the surface of an insect’s exoskeleton and serve as the entry and exit points for gases in the gas exchange system. They are connected to the tracheal system, allowing for the exchange of gases between the environment and the insect’s body.
The number and placement of spiracles vary among different insect species. Typically, they are located on the thorax and abdomen, with pairs of spiracles on each body segment. The arrangement of spiracles ensures widespread oxygen intake and efficient removal of carbon dioxide.
Spiracles can be opened and closed by specialized muscles, giving insects the ability to regulate the flow of gases. By controlling the opening and closing of spiracles, insects can prevent excessive water loss and limit the entry of unwanted particles or toxins. The ability to selectively open and close spiracles is essential for the survival of insects in different environments and helps maintain their internal homeostasis.
In some insects, certain spiracles are modified to serve specific functions. For example, in aquatic insects, spiracles located on the abdomen may be equipped with gills or plastrons. These structures enable gas exchange to occur in water environments, allowing the insects to extract oxygen from water while minimizing water loss through their respiratory system.
Spiracles also play a role in the release of excess water vapor. Insects have a higher surface area-to-volume ratio than mammals, making them more prone to water loss. By opening spiracles, insects can rid their bodies of excess water vapor, a process known as transpiration. Through the regulation of spiracle opening, insects maintain water balance and prevent dehydration.
It is important to note that the process of gas exchange in insects is largely passive and relies on diffusion. Oxygen moves from areas of higher concentration (the external environment) to areas of lower concentration (the cells within the insect’s body), while carbon dioxide follows the opposite direction. Spiracles and the tracheal system facilitate this diffusion process, ensuring vital gas exchange throughout an insect’s body.
Overall, spiracles are essential structures in the gas exchange system of insects. Their ability to open and close, their distribution across the body, and their modifications in certain species allow insects to adapt to diverse environments and maintain efficient oxygen uptake while minimizing water loss.
3 Tracheoles
Tracheoles are tiny tubes that branch off from the tracheae in the gas exchange system of insects. These microscopic structures play a crucial role in delivering oxygen directly to the cells and removing carbon dioxide.
Tracheoles are highly specialized for gas exchange. They have extremely thin walls, often just one cell layer thick, and are in close proximity to individual cells. This proximity allows for efficient diffusion of gases, ensuring the rapid exchange of oxygen and carbon dioxide.
When an insect is in an active state and requires a higher rate of oxygen uptake, the tracheoles can extend to reach the most metabolically active tissues. This branching network of tracheoles ensures that every cell has access to oxygen, regardless of its location in the insect’s body. In contrast, during periods of rest or reduced oxygen demand, the tracheoles can retract or collapse, conserving energy and reducing unnecessary surface area for gas exchange.
Tracheoles penetrate into the cells through tiny invaginations called tracheal pits. These pits are areas where the cell membrane folds inwards, allowing for a closer association between the tracheole and the cell. This close proximity of the tracheole to the cell membrane further facilitates efficient diffusion of gases, ensuring that oxygen quickly reaches the mitochondria, where it is used for cellular respiration.
Due to their small size and the direct contact with cells, tracheoles also play a role in removing waste products, such as carbon dioxide, from the cells. As carbon dioxide diffuses out of the cells, it enters the tracheoles and is transported back to the tracheae, where it is expelled from the body through the spiracles.
The presence of a dense network of tracheoles provides insects with a remarkable respiratory adaptation that allows for efficient gas exchange at the cellular level. The proximity of tracheoles to cells, their ability to extend and retract, and their role in removing waste products all contribute to the overall efficiency of the insect respiratory system.
Gas Exchange Mechanism in Insects
The gas exchange mechanism in insects primarily relies on diffusion, a passive process by which gases move from areas of higher concentration to areas of lower concentration. This mechanism ensures that oxygen is delivered to the cells and carbon dioxide is removed from the body.
As insects do not have specialized respiratory organs like lungs, their entire body surface participates in gas exchange. Oxygen enters the body through the spiracles, which are connected to the tracheal system. From the spiracles, oxygen diffuses through the tracheae and into the finer tracheoles, which penetrate the cells. In the cells, oxygen is used in cellular respiration, and carbon dioxide is produced as a waste product.
Diffusion in insects occurs due to concentration gradients. Oxygen, which is higher in concentration in the external environment, moves into the body where its concentration is lower. Similarly, carbon dioxide, which is higher in concentration within the cells, diffuses out of the cells and into the tracheoles and tracheae, where its concentration is relatively lower.
The efficiency of gas exchange in insects is enhanced by several factors. Firstly, the extensive branching of the tracheal system ensures that oxygen can reach even the deepest tissues in the body. Secondly, the thin walls of the tracheoles and the close proximity to cells facilitate rapid diffusion of gases. The high surface area-to-volume ratio of insect bodies further aids in efficient gas exchange.
In addition to diffusion, the movement of gases within the tracheal system also contributes to gas exchange in insects. This movement is facilitated by changes in pressure brought about by muscle contractions. Muscular contractions within the body can compress the tracheae, forcing air to move from regions of higher pressure to regions of lower pressure. This pumping action helps to ventilate the tracheal system, ensuring a fresh supply of oxygen and the removal of carbon dioxide.
A combination of diffusion and pressure changes enables insects to maintain a constant supply of oxygen to their cells and remove carbon dioxide efficiently. The compact and decentralized gas exchange mechanism in insects ensures that every cell in their small bodies receives the oxygen it needs for metabolic processes.
1 Diffusion
Diffusion is a critical process in the gas exchange mechanism of insects. It is the movement of gases from an area of high concentration to an area of low concentration, driven by concentration gradients.
In insects, diffusion occurs at multiple levels—between the external environment and the tracheal system, within the tracheal system, and between the tracheoles and the cells.
At the first level, oxygen enters the insect’s body through the spiracles, which are connected to the tracheal system. The spiracles open, allowing oxygen to flow into the tracheae. Since the concentration of oxygen is higher in the environment than in the tracheae, it diffuses down the concentration gradient and enters the tracheal system.
Within the tracheal system, diffusion continues as oxygen moves from the larger tracheae to the smaller tracheoles. The concentration of oxygen decreases as it moves deeper into the system. This concentration gradient facilitates the diffusion of oxygen into the tracheoles and closer to the cells.
At the cellular level, oxygen diffuses from the tracheoles into the cells, where it is utilized in cellular respiration. The cells consume oxygen and release carbon dioxide as a waste product. The higher concentration of carbon dioxide in the cells compared to the tracheoles favours its diffusion from the cells into the tracheoles and back into the tracheal system.
The thin walls of the tracheoles and their close proximity to cells enhance the efficiency of diffusion. These characteristics reduce the diffusion distance and allow for a rapid exchange of gases between the tracheoles and the cells. This ensures that oxygen can quickly reach the mitochondria, where it is utilized for energy production, and that carbon dioxide can be efficiently removed from the cells.
Diffusion plays a crucial role in the gas exchange mechanism of insects. It enables the passive movement of gases, ensuring the delivery of oxygen to cells and the removal of carbon dioxide. The efficient diffusion between the tracheal system and the cells allows insects to meet their metabolic needs, despite not having specialized respiratory organs like lungs.
2 Movement of Gases
In addition to diffusion, the movement of gases within the tracheal system is an important aspect of gas exchange in insects. This movement is driven by changes in pressure created by various mechanisms, including muscular contractions and changes in body position.
One of the primary mechanisms of gas movement in insects is through muscle contractions. Insects possess specialized muscles associated with the tracheal system and spiracles. These muscles can contract and relax, causing changes in the volume of the tracheal tubes. When the muscles contract, the tracheal tubes are squeezed, resulting in an increase in pressure. This increase in pressure pushes the air along the tracheal system and aids in the movement of gases.
Local contractions of muscles near certain spiracles can also help regulate gas flow. By contracting specific muscles, insects can close off or open specific spiracles, allowing them to control the direction and amount of air flow. This selective control of spiracles is crucial for preventing water loss, regulating gas exchange rates, and protecting against environmental pollutants.
In some insects, wing movements also aid in gas exchange. For instance, in flying insects, the beating of wings causes rapid vibrations, leading to an increase in air flow across the spiracles. This increased flow facilitates the exchange of gases and helps replenish oxygen levels during intense flight activity.
Other factors, such as body movements and changes in body position, can also influence gas movement in insects. For example, some insects may perform abdominal contractions or expand and contract their thoracic muscles, which assist in ventilating the tracheal system. In certain situations, insects may alter their body position to maximize gas exchange, such as extending their abdomen or extending and flexing their abdomen in a pumping motion.
The movement of gases within the tracheal system ensures a continuous supply of oxygen to the cells and the removal of carbon dioxide. By utilizing muscle contractions and other mechanisms, insects can actively control gas flow and optimize gas exchange depending on their metabolic demands and environmental conditions.
In summary, the movement of gases within the tracheal system of insects complements the diffusion process, promoting the efficient exchange of oxygen and carbon dioxide. Muscle contractions, wing movements, body position adjustments, and selective control of spiracles all contribute to maintaining a constant supply of oxygen and removing waste gases, enabling insects to thrive in diverse environments.
Compare and Contrast with Mammalian Gas Exchange System
The gas exchange systems of insects and mammals differ significantly in terms of their respiratory organs, lung structure, and breathing mechanism.
One of the most notable differences between the two systems is the presence of specialized respiratory organs in mammals, namely the lungs. Mammalian lungs are complex structures consisting of millions of tiny air sacs called alveoli. These alveoli provide a large surface area for gas exchange to occur, allowing for efficient oxygen uptake and carbon dioxide release.
In contrast, insects lack lungs and instead rely on a decentralized system of tubes known as the tracheal system. The tracheal system directly delivers oxygen to the cells via an extensive network of tracheae and tracheoles, allowing for direct gas exchange without the need for a circulatory system. This decentralized system enables insects to rapidly supply oxygen to all cells, regardless of the distance from the external environment.
Another difference lies in the structure of the respiratory surfaces. In mammalian lungs, the alveoli are thin-walled structures lined with capillaries, providing a large surface area for efficient gas exchange. Insects, on the other hand, have highly branched tracheoles that directly come into contact with individual cells, ensuring oxygen delivery to every tissue.
The breathing mechanisms of insects and mammals also differ. Mammals rely on muscular contractions of the diaphragm and intercostal muscles to expand and contract the lungs, creating a pressure gradient that allows air to flow in and out. This process is known as tidal breathing, where air moves in and out of the lungs through a centralized pathway.
In contrast, insects do not have a centralized respiratory pathway like mammals. Instead, they rely on the diffusion of gases in and out of the tracheal system. Additionally, insects can actively control the movement of air within the tracheal system through muscular contractions and adjustments in body position, facilitating efficient gas exchange.
Despite these differences, both insects and mammals share the common goal of oxygen uptake and carbon dioxide removal to support cellular respiration. While mammals have evolved lungs as specialized respiratory organs, insects have developed a decentralized and highly efficient tracheal system.
In summary, the gas exchange systems of insects and mammals vary in their respiratory organs, lung structure, and breathing mechanisms. Mammals utilize lungs and tidal breathing, while insects rely on the tracheal system and diffusion. These adaptations highlight the remarkable diversity seen in the animal kingdom’s respiratory strategies, allowing organisms to thrive in different environments and meet their metabolic demands.
1 Respiratory Organs
The respiratory organs in insects and mammals differ significantly in their structure and function. Mammals have specialized respiratory organs known as lungs, while insects lack lungs and rely on a decentralized system for gas exchange.
In mammals, the lungs are the primary respiratory organs responsible for the exchange of gases. They are large, elastic structures located within the thoracic cavity. The lungs contain millions of tiny air sacs called alveoli, which provide a vast surface area for the exchange of oxygen and carbon dioxide.
The alveoli in mammalian lungs are lined with a thin layer of cells and surrounded by an extensive network of capillaries. This arrangement allows for efficient gas exchange, as oxygen from inhaled air diffuses into the bloodstream, while carbon dioxide generated during cellular respiration moves from the blood into the alveoli to be exhaled.
In contrast, insects lack specialized respiratory organs like lungs. Instead, they possess a unique system of gas exchange called the tracheal system. The tracheal system consists of a network of tubes called tracheae, which extend throughout the insect’s body.
The tracheae in insects are reinforced with chitin, a rigid substance found in their exoskeletons. This reinforcement provides support and prevents the collapse of the tracheal tubes. The tracheae branch extensively, reaching all parts of the insect’s body, ensuring oxygen delivery even to the most remote tissues.
While mammals rely on a centralized system (lungs) to transport gases, insects have a decentralized system. The tracheae in insects directly deliver oxygen to the cells through smaller tubes called tracheoles, which penetrate into individual cells. This direct delivery allows for efficient gas exchange without the need for an elaborate circulatory system.
Overall, mammals and insects employ different respiratory organs to support gas exchange. Mammals have complex lungs with alveoli for efficient oxygen uptake and carbon dioxide release. In contrast, insects possess a decentralized tracheal system that directly delivers oxygen to cells. Despite these differences, both respiratory systems have evolved to meet the unique metabolic demands of each group, ensuring the supply of oxygen for cellular respiration.
2 Lung Structure
The structure of lungs, the respiratory organs in mammals, is complex and specifically adapted for efficient gas exchange. Lungs consist of a branching network of airways that lead to millions of tiny air sacs called alveoli.
The structure of the lung is designed to maximize the surface area available for gas exchange. The airways within the lung, including the bronchi, bronchioles, and terminal bronchioles, progressively divide into smaller branches, eventually culminating in the respiratory bronchioles and alveolar ducts.
The respiratory bronchioles connect to clusters of alveoli, which are tiny air sacs lined with a single layer of epithelial cells. The walls of the alveoli are extremely thin, allowing for efficient diffusion of gases. Surrounding the alveoli are an extensive network of capillaries, where oxygen and carbon dioxide are exchanged between the air and the bloodstream.
The alveolar walls are thin and moist, facilitating the diffusion of oxygen from the air within the alveoli into the bloodstream. Similarly, carbon dioxide, a waste product of cellular respiration, diffuses from the bloodstream into the alveoli to be exhaled from the body.
The structure of the lung also includes important features that aid in gas exchange. One of these features is the presence of surfactant, a lipid substance that reduces surface tension within the alveoli. Surfactant prevents the collapse of alveoli during exhalation and helps maintain their stability for efficient gas exchange.
Furthermore, the lungs are highly vascularized, meaning they have a rich supply of blood vessels. The capillaries surrounding the alveoli have thin walls, allowing for efficient gas exchange between the air and the bloodstream. This close proximity between the respiratory surface and the blood vessels ensures a rapid exchange of oxygen and carbon dioxide.
The structure of lungs in mammals is specialized to optimize gas exchange. The branching airways and alveoli provide a large surface area for efficient diffusion of gases. The thin and moist alveolar walls, along with the extensive capillary network, ensure the rapid exchange of oxygen and carbon dioxide, supporting cellular respiration.
3 Breathing Mechanism
The breathing mechanism in mammals, including humans, involves the coordinated effort of the diaphragm, intercostal muscles, and other accessory muscles to facilitate the movement of air into and out of the lungs.
During inhalation, the diaphragm, a dome-shaped muscle located at the base of the chest cavity, contracts and flattens. This downward movement increases the volume of the chest cavity, causing a decrease in its internal pressure. Simultaneously, the external intercostal muscles contract, lifting the ribcage and further expanding the chest cavity.
As the chest cavity expands, the pressure within the lungs decreases, creating a pressure gradient between the lungs and the external environment. This gradient causes air to flow from an area of higher pressure (the atmosphere) to an area of lower pressure (the lungs), resulting in inhalation.
During exhalation, the diaphragm and intercostal muscles relax, allowing the chest cavity to return to its resting position. This reduction in volume increases the pressure within the lungs, causing air to be expelled from the lungs to equalize the pressure gradient.
The breathing mechanism in mammals follows a pattern of inhalation and exhalation, known as tidal breathing. It is a continuous process that occurs involuntarily, driven by the respiratory centers in the brain stem and influenced by factors such as oxygen and carbon dioxide levels in the blood.
Contrastingly, insects do not have a centralized respiratory system like mammals, and their breathing mechanism differs significantly. Insects rely mainly on passive mechanisms and movement of gases facilitated by changes in pressure.
Insects lack diaphragms or other specialized respiratory muscles. Instead, they rely on the movement of gases through their tracheal system via diffusion. Oxygen enters the tracheae through the spiracles, driven by concentration gradients, and reaches cells through the tracheoles. Similarly, carbon dioxide diffuses from the cells into the tracheoles and is expelled through the spiracles.
Insects can also actively regulate gas flow by opening and closing the spiracles using specialized muscles. This allows them to control the direction and amount of air entering and leaving the tracheal system, helping to prevent excessive water loss and regulate gas exchange rates.
The breathing mechanisms in mammals and insects are fundamentally different. Mammals employ muscular contractions to expand and contract the lungs, creating a pressure gradient that drives the movement of air. In contrast, insects rely on passive mechanisms and diffusion within their tracheal system, aided by selective control of spiracles to regulate gas flow.
Adaptations of Insect Gas Exchange System
The gas exchange system of insects is characterized by several remarkable adaptations that allow them to meet their oxygen demands efficiently while conserving water and maintaining a rapid gas exchange rate.
One key adaptation of the insect gas exchange system is the decentralized tracheal system. This system delivers oxygen directly to cells via tracheae and tracheoles, eliminating the need for a circulatory system to transport gases. The extensive branching of the tracheal system ensures that oxygen can reach all cells, even in insects with complex body shapes. This decentralization enables rapid and direct oxygen delivery, enhancing metabolic efficiency.
In addition, the tracheal system allows insects to conserve water. Unlike mammalian lungs, which require moist surfaces for gas exchange, insects have a low surface area-to-volume ratio, reducing the risk of desiccation. Moreover, insects can regulate water loss by opening and closing spiracles. By controlling the rate of gas exchange and minimizing water loss, insects can survive in diverse environments, including arid conditions.
Furthermore, the tracheal system enables insects to have a rapid gas exchange rate. Oxygen diffuses directly to cells through the tracheoles, which have thin walls and are in close proximity to individual cells. This efficient diffusion pathway shortens the distance for gases to travel, facilitating quick gas exchange. This adaptation is crucial for the high metabolic demands of insects, which often exhibit rapid movements and a high rate of energy expenditure.
Insects also possess specific adaptations to maximize oxygen uptake. For example, some insects have specialized structures known as plastrons, which allow them to extract oxygen from water environments. Plastrons are thin layers of air held against the body surface by hydrophobic hairs. They create an oxygen-rich microenvironment, enabling the insects to respire in aquatic habitats.
Additionally, certain insects have evolved anatomical features that enhance respiration. For instance, some beetle species have expanded tracheae called air sacs, which can act as oxygen reservoirs. These air sacs help maintain a continuous supply of oxygen during periods of increased oxygen demand, such as during flight or strenuous activities.
The adaptations of the insect gas exchange system highlight the incredible diversity and efficiency of respiratory strategies among different organisms. From the decentralized tracheal system to water conservation mechanisms and specialized structures for oxygen uptake, insects have evolved a variety of adaptations that enable them to thrive in diverse environments and meet their metabolic demands.
1 Efficient Oxygen Delivery
Efficient oxygen delivery is crucial for the survival of insects, as their small size and high metabolic rate demand a rapid and effective means of supplying oxygen to all cells. The adaptations in the insect gas exchange system allow for efficient oxygen delivery throughout their bodies.
One key adaptation is the extensive branching of the tracheal system. The tracheae distribute oxygen throughout an insect’s body, ensuring that even the deepest tissues receive a sufficient supply of oxygen. This branching allows for a highly efficient network that can deliver oxygen directly to every cell, regardless of its location.
The tracheal system’s direct delivery of oxygen eliminates the need for a circulatory system to transport gases, making oxygen uptake quicker and more efficient. Unlike in mammals, where oxygen must be transported via the bloodstream, insects have a decentralized system that delivers oxygen directly to the cells without the delay of transportation.
The tracheoles, the finest branches of the tracheal system, play a crucial role in efficient oxygen delivery. These small tubes penetrate into individual cells, bringing oxygen into close proximity with mitochondria, the powerhouses of the cell. This direct delivery eliminates diffusion barriers and allows for rapid oxygen uptake for cellular respiration.
Moreover, the tracheal system in insects can actively regulate gas flow through the opening and closing of spiracles. This selective control allows insects to direct oxygen to specific areas that require more oxygen, such as active flight muscles. By regulating the flow of oxygen, insects can efficiently supply oxygen to tissues with higher metabolic demands, enhancing their overall performance.
Insects also exhibit adaptations that enable efficient oxygen delivery during periods of increased demand. Some insects have specialized air sacs or expanded tracheae that act as oxygen reservoirs. These adaptations allow insects to store extra oxygen and release it when there is a sudden spike in oxygen demand, such as during intense flight or rapid locomotion.
Overall, the adaptations in the insect gas exchange system, such as the extensive branching of the tracheal system, direct delivery of oxygen, selective control of gas flow, and specialized oxygen storage mechanisms, ensure efficient and adequate oxygen delivery to every cell. These adaptations allow insects to maintain their high metabolic rate, supporting their energetic needs and enabling them to thrive in various ecological niches.
2 Conservation of Water
Water conservation is a vital aspect of the insect gas exchange system, as insects are often exposed to arid environments where water availability is limited. Their adaptations allow them to minimize water loss while still efficiently exchanging gases.
One key adaptation for water conservation is the ability to selectively open and close spiracles, the openings in the exoskeleton that allow for gas exchange. By controlling the opening and closing of spiracles, insects can minimize water loss through transpiration. In arid conditions or when water availability is low, insects can close their spiracles to reduce the rate of water evaporation from their bodies.
In addition to spiracle control, the presence of chitin, a component of the insect exoskeleton, plays a role in water conservation. Chitin is a waterproof substance that helps retain moisture within the insect’s body, minimizing water loss through the cuticle. This adaptation is particularly important for insects that inhabit dry environments.
Some insects have evolved specialized structures to further conserve water. For instance, certain desert-dwelling insects have hairs or scales on their body surface, which create a microenvironment that traps moisture and reduces evaporation. These adaptations help them retain water and survive in their arid habitats.
Furthermore, the structure of the tracheal system itself contributes to water conservation. The air-filled tracheal tubes do not require a moist environment for gas exchange, unlike mammalian lungs. This reduces the risk of water loss during respiration.
Additionally, insects exhibit behavioral adaptations that aid in water conservation. For example, many insects are active during cooler and more humid periods of the day, such as early morning or evening. This allows them to reduce water loss through transpiration by avoiding the hottest and driest parts of the day.
In summary, the insect gas exchange system has numerous adaptations that enable efficient gas exchange while conserving water. The ability to selectively open and close spiracles, the presence of a waterproof cuticle, specialized structures for retaining moisture, and behavioral adaptations all contribute to the efficient use of water resources by insects. These adaptations help them survive in arid conditions and environments with limited water availability, ensuring their physiological functions are sustained while minimizing water loss.
3 Rapid Gas Exchange Rate
Insects possess a gas exchange system that allows for a rapid exchange of gases, ensuring efficient oxygen uptake and carbon dioxide removal. Their adaptations enable them to maintain a high metabolic rate and support their energetic needs.
One key adaptation for rapid gas exchange is the direct delivery of oxygen to all cells through the tracheal system. The extensive branching of the tracheae ensures that oxygen can quickly reach every tissue, regardless of its location in the insect’s body. This direct delivery system eliminates the need for oxygen to be transported through the bloodstream, reducing diffusion distances and facilitating high oxygen delivery rates.
The tracheoles, which are the finest branches of the tracheal system, play a crucial role in facilitating rapid gas exchange. Their thin walls and close proximity to cells allow for efficient diffusion of gases. Oxygen rapidly diffuses from the tracheoles into the cells, while carbon dioxide, a waste product of cellular respiration, diffuses from the cells into the tracheoles to be expelled.
Insects also exhibit adaptations that enhance gas exchange rates during periods of increased demand. Some insects have specialized structures, such as air sacs or expanded tracheae, that act as oxygen reservoirs. These reservoirs store extra oxygen and release it when there is a sudden surge in oxygen demand, such as during flight or intense physical activity.
Moreover, insect gas exchange is aided by the ability to actively control gas flow through the opening and closing of spiracles. By selectively opening spiracles in regions that require a higher oxygen supply, such as during intense muscle activity, insects can optimize gas exchange rates and ensure an adequate supply of oxygen to meet increased metabolic demands.
Additionally, the decentralized nature of the insect gas exchange system contributes to rapid gas exchange. With a vast network of tracheae reaching deep into the body, oxygen can be delivered quickly and directly to cells without the need for the coordination of a centralized organ.
Overall, the adaptations in the insect gas exchange system enable rapid gas exchange rates. The direct delivery of oxygen, efficient diffusion through the tracheoles, specialized oxygen reservoirs, and selective control of spiracles all play a role in maintaining a high metabolic rate and supporting the energetic demands of insects. These adaptations ensure that insects can sustain their rapid gas exchange rates, facilitating their diverse range of activities and lifestyles.
Conclusion
The gas exchange system in insects is a remarkable example of adaptation and efficiency. Insects have evolved unique respiratory mechanisms to meet their oxygen demands and ensure efficient removal of carbon dioxide. Through the decentralized tracheal system, insects are able to directly deliver oxygen to every cell, eliminating the need for specialized respiratory organs like lungs found in mammals. This direct delivery system, along with the extensive branching of the tracheae and the close proximity of the tracheoles to cells, allows for rapid gas exchange rates.
Insects have also developed adaptations that allow for efficient oxygen delivery and conservation of water. Their ability to selectively open and close spiracles helps minimize water loss and regulate gas exchange rates. The presence of chitin in their exoskeletons and specialized structures like plastrons and scales further aid in water conservation. These adaptations enable insects to survive in diverse environments, including arid habitats, where water availability is limited.
The rapid gas exchange rate of insects is facilitated by factors such as tracheal branching, direct delivery of oxygen, efficient diffusion through tracheoles, and specialized oxygen reservoirs. These adaptations allow insects to maintain their high metabolic rates, participate in vigorous activities, and thrive in their respective ecological niches.
Understanding the gas exchange system in insects not only provides insights into their fascinating adaptations but also highlights the complexity of respiratory mechanisms in the animal kingdom. The comparison with the mammalian gas exchange system emphasizes the diverse strategies that organisms have evolved to meet their physiological needs in different environments.
The study of insect gas exchange has practical implications as well. Insect respiration plays a vital role in insect physiology, behavior, and ecological interactions. It also has implications for pest control strategies and the development of more efficient insect-inspired technologies.
In summary, the gas exchange system in insects is a remarkable and intricate adaptation that enables efficient oxygen uptake, carbon dioxide release, and water conservation. The decentralized tracheal system, efficient diffusion mechanisms, and selective control of spiracles contribute to the success and survival of insects in diverse habitats and allow them to thrive in their unique ecological roles.