Home>Gardening Basics>Understanding Soil>How Does Nitrogen Get Into The Soil
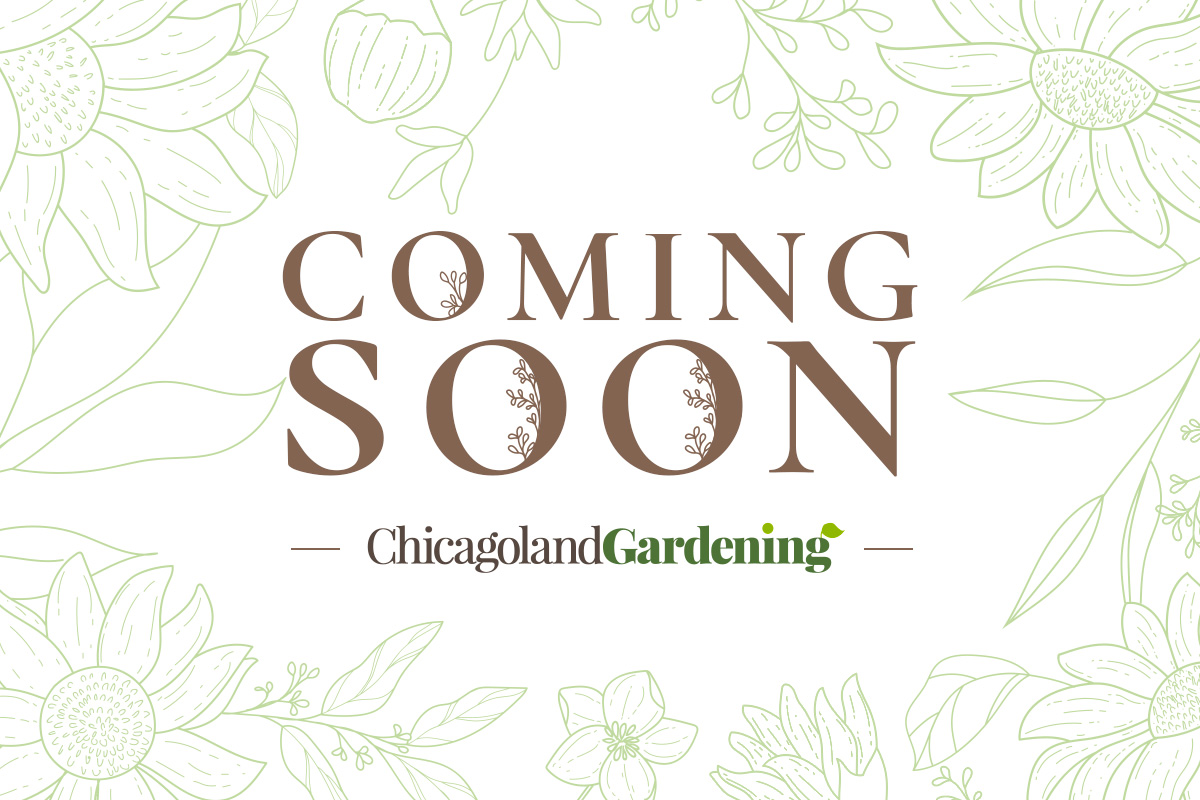
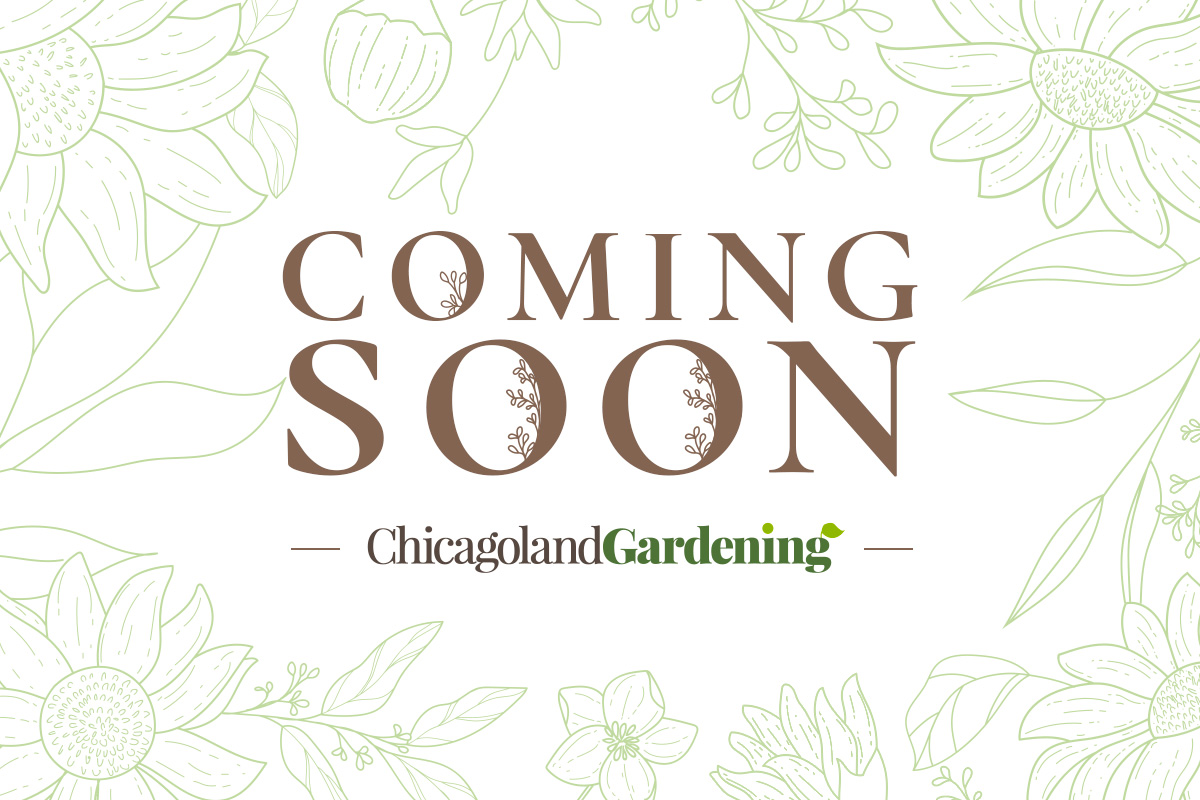
Understanding Soil
How Does Nitrogen Get Into The Soil
Published: February 8, 2024
Learn how nitrogen enters the soil and the role of understanding soil in this process. Explore the importance of nitrogen in soil health and fertility.
(Many of the links in this article redirect to a specific reviewed product. Your purchase of these products through affiliate links helps to generate commission for Chicagolandgardening.com, at no extra cost. Learn more)
Table of Contents
Introduction
Soil is a precious natural resource that sustains life on Earth. It provides the foundation for plant growth, acts as a reservoir for water and nutrients, and supports the intricate web of life in ecosystems. Understanding the processes that occur in the soil is essential for maximizing agricultural productivity, managing environmental impact, and ensuring the long-term health of our planet.
Among the essential nutrients needed by plants, nitrogen plays a vital role in their growth and development. Nitrogen is a key component of proteins, DNA, and other important biomolecules. It is necessary for photosynthesis, energy metabolism, and the synthesis of secondary metabolites. While nitrogen is abundant in the atmosphere, it needs to be converted into a usable form in the soil before plants can uptake it.
In this article, we will explore the different sources of nitrogen in the soil and how it becomes available for plant uptake. We will also discuss the effects of nitrogen on soil health and the environment. Let’s dive deeper into the fascinating world of nitrogen and its role in soil fertility.
Sources of Nitrogen in the Soil
Nitrogen in the soil originates from various natural and anthropogenic sources. Understanding these sources is crucial for managing nutrient availability and ensuring sustainable agriculture. Let’s explore the primary sources of nitrogen in the soil.
1. Biological Fixation: One of the major sources of nitrogen in the soil is biological fixation. Certain bacteria, known as nitrogen-fixing bacteria, have the ability to convert atmospheric nitrogen into a form that plants can use. These bacteria form symbiotic associations with leguminous plants, such as peas and soybeans, or can also be free-living in the soil. Through a process called nitrogen fixation, these bacteria convert atmospheric nitrogen into ammonia, which is then converted into nitrate, a form that plants can readily absorb.
2. Atmospheric Deposition: Nitrogen can also enter the soil through atmospheric deposition. Atmospheric nitrogen compounds, primarily in the form of nitric oxide and ammonia, are released into the air through natural processes like volcanic eruptions and forest fires, as well as human activities like fossil fuel combustion and industrial emissions. These compounds can be transported over long distances and eventually deposited onto the soil surface through rain or dry deposition. Atmospheric deposition is particularly significant in areas close to urban centers or industrial activities.
3. Organic Matter Decomposition: Another important source of nitrogen in the soil is the decomposition of organic matter. When plants and animals die or shed their leaves, the organic material breaks down, releasing nitrogen back into the soil. This process is facilitated by microorganisms, such as bacteria and fungi, that break down complex organic compounds into simpler forms. The nitrogen released during decomposition becomes available for plants to utilize.
4. Nitrogen Fertilizers: The use of nitrogen fertilizers in agriculture is a significant source of nitrogen in the soil. Nitrogen fertilizers provide readily available nitrogen to crops, promoting their growth and enhancing yields. These fertilizers can be inorganic, such as ammonium nitrate or urea, or organic, such as compost or manure. While nitrogen fertilizers contribute to higher crop productivity, their excessive or improper use can lead to environmental issues like water pollution and soil degradation.
5. Industrial Pollution: Industrial activities, such as the burning of fossil fuels, can release nitrogen compounds into the atmosphere, which eventually find their way into the soil through deposition. Industrial pollution can significantly increase nitrogen levels in the soil, leading to environmental imbalances and detrimental effects on ecosystems.
Understanding the sources of nitrogen in the soil allows us to assess nutrient availability, implement appropriate fertilizer management practices, and mitigate the adverse effects of nitrogen pollution. Now that we have explored the sources of nitrogen, let’s delve into the effects it has on soil health and the environment.
Biological Fixation
Biological fixation is a natural process by which certain types of bacteria convert atmospheric nitrogen into a form that plants can use. This process is vital for maintaining nitrogen levels in the soil and promoting plant growth. Let’s take a closer look at how biological fixation occurs and its significance in sustainable agriculture.
Certain bacteria, known as nitrogen-fixing bacteria, have a unique ability to convert atmospheric nitrogen (N₂) into ammonia (NH₃), a form that can be readily utilized by plants. These bacteria form symbiotic associations with leguminous plants, such as peas, beans, and clovers. In these associations, the bacteria reside in nodules on the plants’ roots, supplying them with nitrogen in exchange for carbohydrates and other nutrients. Additionally, some nitrogen-fixing bacteria are free-living in the soil, without the need for a symbiotic relationship with plants.
Nitrogen fixation is a complex process that requires the enzyme nitrogenase, which is produced by the nitrogen-fixing bacteria. This enzyme allows the bacteria to break the strong triple bond between the nitrogen molecules in the atmosphere and convert it into a form that can be utilized by plants. The bacteria capture atmospheric nitrogen and convert it into ammonia, which can further be converted into nitrate (NO₃⁻), a form of nitrogen that plants can take up through their roots.
The significance of biological fixation in sustainable agriculture cannot be overstated. It provides a natural and renewable source of nitrogen for plant growth, reducing the reliance on synthetic fertilizers. This improves soil fertility, reduces input costs for farmers, and minimizes the environmental impacts associated with fertilizer production and application.
In addition to leguminous plants, certain non-leguminous plants can also form associations with nitrogen-fixing bacteria. Some grasses and cereal crops, such as corn and wheat, can develop symbiotic relationships with specific nitrogen-fixing bacteria, called diazotrophic bacteria. These associations can contribute to nitrogen fixation in non-legume crops, further enhancing nutrient availability in agricultural systems.
It’s worth noting that while biological fixation is an important natural process, it may not always provide sufficient nitrogen for all crops or in all agricultural systems. In some cases, supplemental nitrogen from other sources, such as organic matter or nitrogen fertilizers, may be necessary to meet the nutritional requirements of plants.
Understanding biological fixation and its role in nitrogen cycling is essential for sustainable agricultural practices. By utilizing nitrogen-fixing plants or implementing methods to promote the growth of nitrogen-fixing bacteria in the soil, farmers can reduce the need for synthetic fertilizers, enhance soil fertility, and contribute to a more sustainable and environmentally friendly approach to crop production.
Atmospheric Deposition
Atmospheric deposition is a natural process in which nitrogen compounds are released into the air and eventually deposited onto the soil surface through rain or dry deposition. This process provides an important source of nitrogen to the soil and has both natural and anthropogenic origins. Let’s explore atmospheric deposition and its significance in nutrient cycling and soil fertility.
The atmosphere contains various nitrogen compounds, including nitric oxide (NO) and ammonia (NH₃). These compounds can be produced naturally by processes such as lightning, volcanic eruptions, and biological activity, or they can result from human activities like fossil fuel combustion, industrial emissions, and agricultural practices.
When nitrogen compounds are released into the air, they can be transported over long distances by wind currents. Eventually, they undergo deposition, which can occur in two forms: wet deposition and dry deposition.
Wet deposition: This occurs when nitrogen compounds are incorporated into rain, snow, or fog and then deposited onto the soil. The water acts as a carrier, bringing nitrogen compounds from the atmosphere to the ground. Wet deposition is influenced by factors such as rainfall intensity, atmospheric concentration of nitrogen compounds, and the presence of natural or artificial aerosols that can enhance the process. It is particularly important in regions with high precipitation rates.
Dry deposition: Dry deposition occurs when nitrogen compounds settle onto the soil surface without the involvement of precipitation. This can happen through gravitational settling, diffusion, or deposition onto nearby vegetation. Dry deposition is influenced by factors such as wind speed, aerodynamic properties of surfaces, and the concentration and size of airborne particles. It is important in regions with lower precipitation rates or during dry periods.
The nitrogen deposited onto the soil through atmospheric deposition can have significant effects on soil health and plant productivity. It acts as a natural fertilizer, providing a source of nitrogen that plants can utilize for growth and development. However, excessive nitrogen deposition can also have detrimental effects, leading to nutrient imbalances, soil acidification, and changes in the composition and functioning of soil microbial communities.
It is important to note that the extent of nitrogen deposition can vary geographically. Areas close to urban centers, industrial operations, or regions with high agricultural activity may experience higher nitrogen deposition due to increased emissions. Monitoring and managing nitrogen deposition levels are essential to mitigate potential environmental impacts and maintain sustainable agricultural practices.
Overall, atmospheric deposition is a significant source of nitrogen in the soil, contributing to nutrient cycling and soil fertility. It highlights the interconnectedness of natural and human-driven processes and emphasizes the importance of sustainable practices to minimize excessive nitrogen deposition and its potential ecological consequences.
Organic Matter Decomposition
Organic matter decomposition is a fundamental process in soil ecosystems that releases nitrogen into the soil. When plants and animals die or shed their leaves, their organic material undergoes decomposition, resulting in the breakdown of complex organic compounds into simpler forms. This decomposition process not only contributes to nutrient cycling but also plays a crucial role in maintaining soil fertility. Let’s explore the process of organic matter decomposition and its significance in the nitrogen cycle.
When organic matter, such as dead plants, animal carcasses, or fallen leaves, enters the soil, it becomes a source of nutrients, including nitrogen. Microorganisms, such as bacteria and fungi, play a vital role in breaking down the organic matter. These microorganisms secrete enzymes that help break down complex organic compounds into smaller molecules, including nitrogen-containing compounds.
During decomposition, organic nitrogen compounds, such as proteins and amino acids, are converted into ammonium (NH₄⁺) through a process known as mineralization. Ammonium is then further converted into nitrate (NO₃⁻) through a process called nitrification.
Mineralization involves the release of ammonium from organic matter by soil microorganisms. This ammonium can be directly taken up by plants or transformed into nitrate through nitrification. Nitrification is a two-step process wherein specific bacteria convert ammonium into nitrite (NO₂⁻) and then nitrite into nitrate. Nitrate is the primary form of nitrogen that plants can readily absorb and utilize for growth.
The rate of organic matter decomposition and nitrogen release can be influenced by several factors, including temperature, moisture, pH, oxygen availability, and the quality of organic matter. Warmer temperatures and optimum moisture levels promote faster decomposition and nutrient release. Additionally, soil microorganisms and the presence of decomposers, like earthworms and insects, also affect the decomposition process.
The decomposition of organic matter not only releases nitrogen but also contributes to the overall fertility of the soil. As organic matter decomposes, it improves soil structure, enhances water-holding capacity, promotes nutrient retention, and provides habitat for beneficial soil organisms. It also helps to prevent erosion and contributes to the long-term carbon storage in soils, thus mitigating the effects of climate change.
To optimize organic matter decomposition and nitrogen release in the soil, farmers and gardeners can implement practices like composting, crop residue management, and the addition of organic amendments. These practices not only enhance nutrient cycling but also promote the sustainability and health of agricultural systems.
Understanding the process of organic matter decomposition and its role in nitrogen cycling is crucial for maintaining soil fertility and promoting sustainable agricultural practices. By managing organic inputs and fostering healthy soil ecosystems, we can harness the power of organic matter decomposition to enhance crop productivity while minimizing environmental impacts.
Nitrogen Fertilizers
Nitrogen fertilizers are widely used in agriculture to supplement the nitrogen needs of crops and improve yields. These fertilizers provide readily available nitrogen to plants, promoting their growth and productivity. However, their use requires careful management to ensure optimal nutrient utilization and minimize environmental impacts. Let’s explore nitrogen fertilizers and their importance in agricultural practices.
Nitrogen is an essential nutrient for plant growth and plays a vital role in various physiological processes, including photosynthesis, protein synthesis, and enzyme activity. While nitrogen is naturally present in the soil through organic matter decomposition and biological fixation, it may not always be available in sufficient quantities for crop requirements. Nitrogen fertilizers help bridge this gap by providing readily available nitrogen to plants.
Nitrogen fertilizers come in different forms, including inorganic and organic. Inorganic nitrogen fertilizers are synthetically produced and include compounds such as ammonium nitrate, urea, and ammonium sulfate. These fertilizers contain nitrogen in forms that are easily taken up by plants. Organic nitrogen fertilizers, on the other hand, are derived from natural sources like compost, animal manure, or plant residues. These fertilizers release nitrogen slowly as organic matter decomposes, ensuring a gradual and sustained supply of nutrients to plants.
Applying nitrogen fertilizers to crops can have several benefits. It can increase plant growth and yield, improve crop quality, and enhance nutrient uptake efficiency. Nitrogen allows plants to produce more chlorophyll, resulting in greener and healthier foliage. It also promotes root development, leading to improved nutrient and water uptake. Overall, nitrogen fertilizers help meet the nitrogen demands of high-yielding crops and support sustainable intensification in agriculture.
However, the use of nitrogen fertilizers requires careful management. Excessive or improper application can lead to environmental issues. One of the primary concerns is the potential for nitrogen runoff. When nitrogen fertilizers are applied in excess or during periods of heavy rainfall, the nitrogen can be washed off the fields and enter nearby water bodies. This can contribute to water pollution, promote harmful algal blooms, and disrupt aquatic ecosystems.
Another challenge associated with nitrogen fertilizers is nitrogen leaching. When nitrogen is not taken up by plants or retained in the soil, it can percolate through the soil profile and enter groundwater. This can lead to nitrate contamination of drinking water sources, posing risks to human health, particularly for infants and pregnant women.
Additionally, the overuse of nitrogen fertilizers can result in soil acidification. The application of nitrogen in its nitrate form can increase the acidity of the soil, negatively impacting soil pH and nutrient availability. Soil acidification can hinder nutrient uptake by plants and impair soil microbial activity, leading to reduced soil productivity.
To mitigate these challenges, farmers and agricultural practitioners can adopt best management practices when using nitrogen fertilizers. These practices include proper nutrient management planning, optimizing fertilizer rates and timings, incorporating nitrogen-efficient crop varieties, implementing precision agriculture techniques, and promoting practices that reduce runoff and leaching, such as cover cropping and conservation tillage.
Proper management of nitrogen fertilizers is essential to ensure the efficient use of nutrients, minimize environmental risks, and maintain soil health and productivity. By adopting sustainable nutrient management strategies, we can harness the benefits of nitrogen fertilizers while minimizing their potential drawbacks.
Industrial Pollution
Industrial pollution is a significant source of nitrogen in the soil and can have far-reaching impacts on ecosystems and human health. Industrial activities, such as manufacturing, energy production, and transportation, release nitrogen compounds into the atmosphere, which eventually find their way into the soil through deposition. Let’s explore the effects of industrial pollution on soil nitrogen levels and the environment.
Industrial pollution contributes to elevated nitrogen levels in the soil through the emission of nitrogen compounds, primarily nitric oxide (NO) and ammonia (NH₃). These compounds are released into the air as byproducts of fossil fuel combustion, industrial emissions, and various manufacturing processes. Once released, nitrogen compounds can undergo deposition onto the soil surface through rain or dry deposition.
The effects of industrial pollution on soil nitrogen levels can be both direct and indirect. Direct effects occur when nitrogen compounds in industrial emissions are directly deposited onto the soil, increasing nitrogen availability. Indirect effects result from the atmospheric reactions of nitrogen compounds, which can lead to the formation of secondary pollutants like nitrogen oxides and particulate matter. These secondary pollutants can affect soil pH, nutrient cycling, and overall soil health.
One of the main consequences of industrial pollution is an excessive input of nitrogen into terrestrial ecosystems, a phenomenon known as nitrogen deposition. High levels of nitrogen deposition can disrupt natural nutrient cycling processes, leading to imbalances in the nitrogen cycle. This can have detrimental effects on plant communities, soil microorganisms, and other components of the ecosystem.
Excessive nitrogen deposition can also contribute to soil acidification. Nitrogen compounds, particularly nitric acid derived from nitrogen oxides, can increase soil acidity, reducing pH levels. Soil acidification negatively affects soil fertility and nutrient availability for plants, leading to reduced crop yields and decreased biodiversity.
Moreover, the increased nitrogen availability resulting from industrial pollution can lead to eutrophication, especially in aquatic ecosystems. When nitrogen compounds enter water bodies through runoff or leaching, they can stimulate excessive and rapid algal growth. This algal bloom depletes oxygen levels in the water, which can disrupt aquatic ecosystems and harm fish and other aquatic organisms.
Addressing industrial pollution and its impact on soil nitrogen levels requires robust environmental regulations and sustainable industrial practices. By implementing technologies to reduce emissions, promoting renewable energy sources, and enforcing strict emission standards, we can mitigate the release of nitrogen compounds into the atmosphere. Additionally, implementing proper waste management practices, such as treating industrial effluents and reducing nutrient runoff from industrial sites, can help prevent the contamination of soil and water bodies.
Managing industrial pollution is not only crucial for protecting soil health and biodiversity but also for safeguarding human health. Air pollution caused by industrial activities, including nitrogen compounds, can have adverse respiratory effects on humans, leading to respiratory illnesses and other health complications.
Overall, understanding and addressing the impacts of industrial pollution on soil nitrogen levels and the environment are vital for promoting sustainable industrial development, maintaining soil fertility, and preserving ecosystem functioning and human well-being.
Effects of Nitrogen in the Soil
Nitrogen is a crucial nutrient for plant growth, but excessive or unbalanced levels of nitrogen in the soil can have significant effects on soil health and the environment. Let’s explore the effects of nitrogen in the soil and its implications for agricultural productivity and ecosystem dynamics.
Nitrogen Runoff: Excess nitrogen in the soil can lead to nitrogen runoff, wherein the nitrogen compounds are washed off the fields and enter nearby water bodies. This runoff can occur during heavy rainfall or when nitrogen fertilizers are applied in excess. Nitrogen runoff contributes to water pollution and can lead to the eutrophication of freshwater and marine ecosystems. Eutrophication stimulates excessive algal growth, depleting oxygen levels in the water and negatively impacting aquatic organisms.
Nitrogen Leaching: In addition to runoff, nitrogen in the soil can also leach into groundwater. When nitrogen is not absorbed by plant roots or retained in the soil, it can percolate through the soil profile and contaminate underground water sources. This poses risks to drinking water quality and can lead to health concerns, particularly for vulnerable populations.
Soil Acidification: The application of nitrogen fertilizers or the deposition of nitrogen compounds from industrial pollution can cause soil acidification. Nitrogen in its nitrate form can increase soil acidity, reducing pH levels. Soil acidification can negatively affect plant growth and nutrient availability, impacting crop productivity. It can also alter soil microbial activity, leading to shifts in soil microbial communities and potential imbalances in nutrient cycling processes.
Eutrophication: Nitrogen pollution, whether from agricultural runoff or industrial emissions, can contribute to eutrophication in aquatic ecosystems. The excess nitrogen promotes algal blooms, leading to a rapid increase in algal biomass. As the algae die and decompose, oxygen levels in the water are depleted, causing hypoxic or anoxic conditions that harm fish and other aquatic organisms. Eutrophication can disrupt the balance of ecosystems, leading to biodiversity loss and ecological imbalances.
Changes in Plant Communities: The availability of nitrogen in the soil can influence plant communities. Nitrogen-loving plants, known as nitrophilous plants, have a competitive advantage in nitrogen-rich environments. In contrast, native plant species adapted to low nitrogen conditions may be outcompeted by more aggressive and non-native species, resulting in a decline in biodiversity.
Greenhouse Gas Emissions: Nitrogen in the soil can contribute to greenhouse gas emissions. When nitrogen is present in the form of nitrous oxide (N₂O), it can be released into the atmosphere through microbial processes, particularly denitrification. Nitrous oxide is a potent greenhouse gas that contributes to climate change and ozone depletion.
Managing nitrogen in the soil is crucial for minimizing its negative effects and promoting sustainable agriculture. Implementing best management practices, such as optimized fertilizer application, cover cropping, and crop rotation, can help reduce nitrogen losses and improve nutrient use efficiency. Additionally, promoting agroecosystem diversification and incorporating nitrogen-fixing plants into cropping systems can enhance nitrogen cycling and reduce the reliance on synthetic fertilizers.
Understanding the effects of nitrogen in the soil allows us to make informed decisions to minimize the environmental impacts while maximizing agricultural productivity. By adopting sustainable approaches to nitrogen management, we can build resilient agricultural systems and protect the health and integrity of our ecosystems.
Nitrogen Runoff
Nitrogen runoff is a significant concern in agriculture and can have detrimental impacts on water quality and ecosystem health. When excess nitrogen is present in the soil, it can be washed off the fields and enter nearby water bodies, resulting in nitrogen runoff. Let’s explore the causes, consequences, and mitigation strategies associated with nitrogen runoff.
Causes: Nitrogen runoff primarily occurs during heavy rainfall or when nitrogen-based fertilizers are applied in excess. Rainwater carries nitrogen compounds, such as nitrate and ammonium, from the soil surface into streams, rivers, and lakes. Inadequate nutrient management practices, including improper fertilizer application, poor irrigation practices, and inadequate vegetative cover, can significantly contribute to nitrogen runoff.
Consequences: Nitrogen runoff poses several environmental challenges. When nitrogen reaches water bodies, it promotes the growth of algae and other aquatic plants. The excessive growth of these organisms, known as algal blooms, depletes oxygen levels in the water through the process of eutrophication. This can result in the death of fish and other aquatic organisms due to suffocation. Furthermore, algal blooms can release toxins, affecting the quality of water resources and posing risks to human health.
Mitigation Strategies: Effective management strategies can help mitigate the impacts of nitrogen runoff. Here are a few key approaches:
- Proper nutrient management: Employing precision agriculture techniques, such as site-specific fertilizer application, can help optimize nutrient use and reduce the risk of nitrogen runoff. Applying fertilizers at the right time and in the right amounts, based on soil testing and crop nutrient requirements, can minimize excess nitrogen availability.
- Conservation practices: Implementing soil conservation practices can help reduce runoff and erosion, which indirectly affects the loss of nitrogen. Techniques like contour plowing, terracing, and cover cropping can help maintain soil structure, minimize surface water runoff, and improve water infiltration.
- Riparian buffers: Establishing vegetative buffers, such as trees, shrubs, and grasses along waterways, can act as natural filters, effectively trapping and absorbing nutrients from runoff before they enter water bodies. These riparian buffers also provide habitat for wildlife and can enhance biodiversity.
- Wetlands and constructed wetlands: Wetlands are highly efficient ecosystems for nutrient removal. Constructed wetlands with carefully designed hydraulic characteristics can be used to treat agricultural runoff, effectively reducing nitrogen loads in the water and improving water quality.
- Educational awareness: Educating farmers, landowners, and the general public about the impacts of nitrogen runoff and the importance of adopting sustainable management practices is crucial. Raising awareness about the proper use of fertilizers, nutrient management, and the implementation of conservation practices can help promote responsible agricultural practices and reduce nitrogen runoff.
Reducing nitrogen runoff requires a comprehensive and integrated approach that combines proper nutrient management, conservation practices, and targeted mitigation strategies. By implementing these measures, we can protect water resources, preserve ecosystem health, and promote sustainable agricultural practices.
Nitrogen Leaching
Nitrogen leaching is a process in which excess nitrogen in the soil moves downward through the soil profile and enters groundwater. This can lead to the contamination of drinking water sources and potential ecological consequences. Understanding the causes, impacts, and mitigation strategies associated with nitrogen leaching is essential for sustainable agriculture and environmental protection.
Causes: Nitrogen leaching can occur when the soil contains more nitrogen than the plants can uptake. Factors contributing to nitrogen leaching include excessive fertilizer application, improper timing of fertilizer application, heavy rainfall or irrigation, and soil conditions that promote drainage, such as sandy soils. These conditions can lead to the movement of nitrogen beyond the root zone and into groundwater.
Impacts: Nitrogen leaching can have several adverse effects on both the environment and human health. Here are a few key impacts:
- Water contamination: Nitrate, a common form of nitrogen in soil, is highly soluble in water. When it leaches into groundwater, it can contaminate drinking water sources. Elevated levels of nitrate in drinking water pose health risks, particularly to infants and pregnant women, potentially leading to a condition known as methemoglobinemia or “blue baby syndrome.”
- Eutrophication: Nitrogen leaching contributes to eutrophication in surface waters. As the leached nitrogen reaches rivers, lakes, or coastal areas, it stimulates excessive algal growth, leading to algal blooms. These blooms can result in low oxygen levels in the water, negatively impacting fish and other aquatic organisms, and disrupting ecological balance.
- Loss of nutrient resources: Nitrogen leaching leads to the loss of valuable nitrogen resources for plant growth. When nitrogen is not taken up by crops or retained in the soil, it is effectively lost from the agricultural system, necessitating additional nitrogen inputs to support crop growth and productivity.
Mitigation Strategies: Several strategies can help mitigate nitrogen leaching and reduce its environmental impacts:
- Optimized nutrient management: Implementing precision agriculture techniques, conducting regular soil testing, and adjusting fertilizer application rates can help match nutrient inputs to crop requirements, minimizing excess nitrogen that can leach.
- Cover cropping: Planting cover crops during fallow periods or incorporating them into crop rotations can help scavenge excess nitrogen from the soil. The cover crop can take up the nitrogen, preventing it from leaching into groundwater. After terminating the cover crop, the nitrogen trapped in their biomass can be released and made available for subsequent crops.
- Managed drainage systems: Installing controlled drainage systems or subsurface drainage tiles in fields can help manage excess water and prevent excessive leaching of nitrogen. These systems allow for better control of water table levels, reducing the likelihood of nitrogen moving deeper into the soil.
- Soil conservation practices: Implementing soil erosion control measures, such as contour plowing, terracing, and conservation tillage, can help minimize surface runoff and the movement of nitrogen-laden water into water bodies.
By implementing these mitigation strategies and adopting sustainable agricultural practices, we can reduce the risk of nitrogen leaching, protect water quality, and enhance the long-term sustainability of agricultural systems.
Soil Acidification
Soil acidification is a process in which the pH of the soil decreases, making it more acidic. This can occur naturally over time or be accelerated by human activities, such as excessive fertilizer use or industrial emissions. Soil acidification can have significant implications for soil health, nutrient availability, and overall ecosystem functioning. Let’s explore the causes, impacts, and management strategies associated with soil acidification.
Causes: Soil acidification can have both natural and anthropogenic causes. Natural factors include weathering of minerals, leaching of basic cations, and plant and microbial respiration processes. However, human activities play a significant role in accelerating soil acidification. Excessive use of nitrogen-based fertilizers, such as ammonium-based fertilizers, can release hydrogen ions into the soil, leading to increased soil acidity. Industrial emissions, including sulfur and nitrogen oxides, can also contribute to soil acidification when deposited onto the soil surface through atmospheric deposition.
Impacts: Soil acidification can have several detrimental effects on soil health and plant productivity:
- Nutrient availability: Acidic soils tend to have reduced nutrient availability. The increased acidity can alter the chemical properties of the soil, leading to the leaching and loss of essential cations such as calcium, magnesium, and potassium. This can result in nutrient deficiencies and negatively impact plant growth and development.
- Aluminum and manganese toxicity: Soil acidification can increase the solubility of aluminum and manganese in the soil. At high concentrations, these elements become toxic to plants, impairing root development, nutrient uptake, and overall plant health.
- Microbial activity: Soil acidification can affect the microbial communities in the soil. Acidic conditions can alter the composition and activity of beneficial soil microbes involved in nutrient cycling, organic matter decomposition, and plant-microbe interactions. This can disrupt soil ecosystem functioning and reduce soil fertility.
- Soil structure and permeability: Acidic soils can have detrimental effects on soil structure. Soil aggregates can break down, leading to compacted and poorly aerated soils. Additionally, soil acidification can reduce water infiltration rates and exacerbate the risk of erosion.
Management Strategies: Managing soil acidification requires an integrated approach that focuses on both prevention and remediation:
- Soil testing and balanced fertilization: Regular soil testing helps determine nutrient levels, pH, and any nutrient imbalances. Based on the results, appropriate fertilization strategies can be implemented to maintain optimal nutrient levels and prevent soil acidification. Balanced fertilization with lime or other appropriate soil amendments can help raise pH levels and neutralize soil acidity.
- Liming: Applying agricultural lime helps to increase soil pH and reduce soil acidity. Lime is a common soil amendment that contains calcium and/or magnesium carbonates that neutralize soil acidity over time. The rate of lime application should be determined based on soil type, crop nutrient requirements, and the desired pH level.
- Organic matter addition: Adding organic matter to the soil can help buffer soil acidity and improve soil structure. Organic materials like compost, manure, and plant residues increase cation exchange capacity and enhance nutrient holding capacity, promoting a healthier soil environment.
- Water management: Proper irrigation and water management practices can help reduce soil acidification. Maintaining optimum soil moisture levels and avoiding waterlogged conditions can minimize the leaching of acidic components and nutrient loss.
By adopting these management strategies, farmers and land managers can mitigate the effects of soil acidification, improve nutrient availability, and maintain soil health. Regular monitoring of soil pH, nutrient levels, and fertility is essential for timely interventions and sustainable soil management.
Eutrophication
Eutrophication is a phenomenon characterized by an excessive accumulation of nutrients, particularly nitrogen and phosphorus, in water bodies. This heightened nutrient enrichment leads to accelerated plant growth, especially algae, ultimately disrupting the balance and health of aquatic ecosystems. Understanding the causes, consequences, and management strategies of eutrophication is crucial for mitigating its environmental impacts.
Causes: Eutrophication is primarily caused by the excess input of nutrients, especially nitrogen and phosphorus, into water bodies. The main sources of these nutrients include agricultural runoff, sewage discharge, industrial effluents, and atmospheric deposition. The high nutrient levels, often resulting from human activities, fuel the rapid growth of algae and other aquatic plants.
Consequences: Eutrophication can have severe ecological consequences, including:
- Algal blooms: Elevated nutrient levels stimulate excessive growth of algae and other aquatic plants. These algal blooms give the water a greenish appearance and can lead to a range of problems such as reduced light penetration, oxygen depletion, and the production of harmful toxins.
- Oxygen depletion: As algae die and decompose, bacteria consume the organic matter, consuming large amounts of oxygen. This can lead to oxygen-depleted zones, known as hypoxic or anoxic conditions. In such zones, aquatic organisms such as fish, shellfish, and other aquatic life struggle to survive or may die off, disrupting food chains and reducing biodiversity.
- Loss of habitat: Dense algal blooms and oxygen-depleted areas can lead to detrimental changes in aquatic habitats. The excessive growth of aquatic plants can shadow out submerged vegetation, reducing habitat diversity and negatively impacting other aquatic organisms. This can have cascading effects on the overall health and functioning of the ecosystem.
- Water quality degradation: Eutrophication impairs water quality by reducing clarity, increasing turbidity, and altering chemical parameters such as pH and dissolved oxygen. This deterioration in water quality can affect the aesthetic value of water bodies, limit recreational activities, and impact the availability of clean drinking water.
Management Strategies: To mitigate eutrophication, several management strategies can be implemented:
- Nutrient management: Proper nutrient management, particularly in agriculture, is crucial. Implementing precision farming techniques, optimizing fertilizer practices, and adopting nutrient management plans can help reduce nutrient runoff and minimize the contribution of agricultural activities to eutrophication.
- Wetland construction and restoration: Constructed wetlands can act as natural filters, trapping and cycling nutrients before they reach water bodies. Wetland restoration projects help to reestablish natural nutrient cycling processes and provide habitat for beneficial organisms.
- Riparian buffers: Planting and maintaining vegetative buffer zones along water bodies can help filter and absorb excess nutrients from runoff, reducing the amount of nutrients reaching the water.
- Advanced wastewater treatment: Upgrading existing wastewater treatment facilities to include advanced nutrient removal systems can significantly reduce nutrient inputs into water bodies.
- Public awareness and education: Raising awareness about the impacts of eutrophication and the importance of responsible nutrient management practices is vital for achieving long-term sustainable solutions. Educating communities, stakeholders, and policymakers about the consequences of eutrophication can drive changes in behavior and promote water quality protection.
By implementing these management strategies and adopting sustainable practices, it is possible to reduce nutrient inputs, improve water quality, and restore the health of eutrophied ecosystems. Collaboration among scientists, policymakers, and communities is crucial for the effective management and prevention of eutrophication.
Conclusion
Understanding the complex dynamics of nitrogen in the soil is crucial for sustainable agriculture, environmental stewardship, and the preservation of ecosystem health. From biological fixation to nitrogen fertilizers and the impacts of nitrogen runoff, leaching, soil acidification, and eutrophication, the intricate web of interactions highlights the importance of responsible nutrient management and sustainable practices.
Efficient and targeted use of nitrogen fertilizers, coupled with soil conservation practices, can help optimize nutrient availability for plants while minimizing environmental impacts. Implementing precision agriculture techniques, such as site-specific nutrient application, cover cropping, and crop rotation, helps ensure that nitrogen is used efficiently and effectively.
Managing nitrogen runoff and leaching requires a holistic approach, involving proper water management, soil erosion control measures, and riparian buffers. By minimizing nutrient losses and protecting water quality, we can mitigate the impacts of excessive nitrogen inputs on aquatic ecosystems and human health.
Soil acidification, a consequence of nitrogen-based fertilizers and industrial emissions, requires careful soil monitoring, balanced fertilization, and the strategic use of soil amendments such as lime and organic matter additions. By maintaining optimal pH levels, we can preserve soil fertility and promote nutrient availability for plant growth.
Addressing the challenges of eutrophication necessitates an integrated approach that emphasizes nutrient management, wetland restoration, and advanced wastewater treatment. These measures collectively help reduce nutrient inputs, restore water quality, and protect the delicate balance of aquatic ecosystems.
Recognizing the intricate connections between nitrogen, soil, and the environment empowers us to adopt practices that support sustainable agriculture, preserve soil health, and safeguard the health and integrity of our ecosystems. Effective nutrient management, conservation practices, and public awareness can drive the shift towards a more sustainable future. By working together, we can minimize the adverse effects of nitrogen on the soil and the environment, ensuring a healthy and thriving planet for future generations.